Malaria
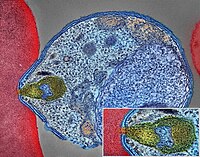
Malaria is a mosquito-borne infectious disease that affects humans and other animals. Malaria causes symptoms that typically include fever, tiredness, vomiting, and headaches. In severe cases, it can cause yellow skin, seizures, coma, or death. Symptoms usually begin ten to fifteen days after being bitten by an infected mosquito. If not properly treated, people may have recurrences of the disease months later. In those who have recently survived an infection, reinfection usually causes milder symptoms. This partial resistance disappears over months to years if the person has no continuing exposure to malaria.
Malaria is caused by single-celled microorganisms of the Plasmodium group. The disease is most commonly spread by an infected female Anopheles mosquito. The mosquito bite introduces the parasites from the mosquito's saliva into a person's blood. The parasites travel to the liver where they mature and reproduce. Five species of Plasmodium can infect and be spread by humans. Most deaths are caused by P. falciparum, whereas P. vivax, P. ovale, and P. malariae generally cause a milder form of malaria. The species P. knowlesi rarely causes disease in humans. Malaria is typically diagnosed by the microscopic examination of blood using blood films, or with antigen-based rapid diagnostic tests. Methods that use the polymerase chain reaction to detect the parasite's DNA have been developed, but are not widely used in areas where malaria is common due to their cost and complexity.
The risk of disease can be reduced by preventing mosquito bites through the use of mosquito nets and insect repellents or with mosquito-control measures such as spraying insecticides and draining standing water. Several medications are available to prevent malaria in travellers to areas where the disease is common. Occasional doses of the combination medication sulfadoxine/pyrimethamine are recommended in infants and after the first trimester of pregnancy in areas with high rates of malaria. As of 2020, there is one vaccine which has been shown to reduce the risk of malaria by about 40% in children in Africa. Efforts to develop more effective vaccines are ongoing. The recommended treatment for malaria is a combination of antimalarial medications that includes artemisinin. The second medication may be either mefloquine, lumefantrine, or sulfadoxine/pyrimethamine. Quinine, along with doxycycline, may be used if artemisinin is not available. It is recommended that in areas where the disease is common, malaria is confirmed if possible before treatment is started due to concerns of increasing drug resistance. Resistance among the parasites has developed to several antimalarial medications; for example, chloroquine-resistant P. falciparum has spread to most malarial areas, and resistance to artemisinin has become a problem in some parts of Southeast Asia.
The disease is widespread in the tropical and subtropical regions that exist in a broad band around the equator. This includes much of sub-Saharan Africa, Asia, and Latin America. In 2018 there were 228 million cases of malaria worldwide resulting in an estimated 405,000 deaths. Approximately 93% of the cases and 94% of deaths occurred in Africa. Rates of disease have decreased from 2010 to 2014 but increased from 2015 to 2017, during which there were 231 million cases. Malaria is commonly associated with poverty and has a significant negative effect on economic development. In Africa, it is estimated to result in losses of US$12 billion a year due to increased healthcare costs, lost ability to work, and adverse effects on tourism.
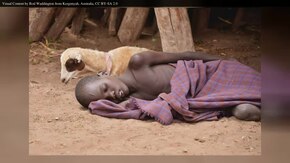
Signs and symptoms

The signs and symptoms of malaria typically begin 8–25 days following infection, but may occur later in those who have taken antimalarial medications as prevention. Initial manifestations of the disease—common to all malaria species—are similar to flu-like symptoms, and can resemble other conditions such as sepsis, gastroenteritis, and viral diseases. The presentation may include headache, fever, shivering, joint pain, vomiting, hemolytic anemia, jaundice, hemoglobin in the urine, retinal damage, and convulsions.
The classic symptom of malaria is paroxysm—a cyclical occurrence of sudden coldness followed by shivering and then fever and sweating, occurring every two days (tertian fever) in P. vivax and P. ovale infections, and every three days (quartan fever) for P. malariae. P. falciparum infection can cause recurrent fever every 36–48 hours, or a less pronounced and almost continuous fever.
Severe malaria is usually caused by P. falciparum (often referred to as falciparum malaria). Symptoms of falciparum malaria arise 9–30 days after infection. Individuals with cerebral malaria frequently exhibit neurological symptoms, including abnormal posturing, nystagmus, conjugate gaze palsy (failure of the eyes to turn together in the same direction), opisthotonus, seizures, or coma.
Complications
Malaria has several serious complications. Among these is the development of respiratory distress, which occurs in up to 25% of adults and 40% of children with severe P. falciparum malaria. Possible causes include respiratory compensation of metabolic acidosis, noncardiogenic pulmonary oedema, concomitant pneumonia, and severe anaemia. Although rare in young children with severe malaria, acute respiratory distress syndrome occurs in 5–25% of adults and up to 29% of pregnant women. Coinfection of HIV with malaria increases mortality. Kidney failure is a feature of blackwater fever, where haemoglobin from lysed red blood cells leaks into the urine.
Infection with P. falciparum may result in cerebral malaria, a form of severe malaria that involves encephalopathy. It is associated with retinal whitening, which may be a useful clinical sign in distinguishing malaria from other causes of fever. An enlarged spleen, enlarged liver or both of these, severe headache, low blood sugar, and haemoglobin in the urine with kidney failure may occur. Complications may include spontaneous bleeding, coagulopathy, and shock.
Malaria in pregnant women is an important cause of stillbirths, infant mortality, miscarriage and low birth weight, particularly in P. falciparum infection, but also with P. vivax.
Cause
Malaria parasites belong to the genus Plasmodium (phylum Apicomplexa). In humans, malaria is caused by P. falciparum, P. malariae, P. ovale, P. vivax and P. knowlesi. Among those infected, P. falciparum is the most common species identified (~75%) followed by P. vivax (~20%). Although P. falciparum traditionally accounts for the majority of deaths, recent evidence suggests that P. vivax malaria is associated with potentially life-threatening conditions about as often as with a diagnosis of P. falciparum infection. P. vivax proportionally is more common outside Africa. There have been documented human infections with several species of Plasmodium from higher apes; however, except for P. knowlesi—a zoonotic species that causes malaria in macaques—these are mostly of limited public health importance.
Life cycle

In the life cycle of Plasmodium, a female Anopheles mosquito (the definitive host) transmits a motile infective form (called the sporozoite) to a vertebrate host such as a human (the secondary host), thus acting as a transmission vector. A sporozoite travels through the blood vessels to liver cells (hepatocytes), where it reproduces asexually (tissue schizogony), producing thousands of merozoites. These infect new red blood cells and initiate a series of asexual multiplication cycles (blood schizogony) that produce 8 to 24 new infective merozoites, at which point the cells burst and the infective cycle begins anew.
Other merozoites develop into immature gametocytes, which are the precursors of male and female gametes. When a fertilised mosquito bites an infected person, gametocytes are taken up with the blood and mature in the mosquito gut. The male and female gametocytes fuse and form an ookinete—a fertilised, motile zygote. Ookinetes develop into new sporozoites that migrate to the insect's salivary glands, ready to infect a new vertebrate host. The sporozoites are injected into the skin, in the saliva, when the mosquito takes a subsequent blood meal.
Only female mosquitoes feed on blood; male mosquitoes feed on plant nectar and do not transmit the disease. Females of the mosquito genus Anopheles prefer to feed at night. They usually start searching for a meal at dusk, and continue through the night until they succeed. Malaria parasites can also be transmitted by blood transfusions, although this is rare.
Recurrent malaria
Symptoms of malaria can recur after varying symptom-free periods. Depending upon the cause, recurrence can be classified as either recrudescence, relapse, or reinfection. Recrudescence is when symptoms return after a symptom-free period. It is caused by parasites surviving in the blood as a result of inadequate or ineffective treatment. Relapse is when symptoms reappear after the parasites have been eliminated from the blood but persist as dormant hypnozoites in liver cells. Relapse commonly occurs between 8–24 weeks and is often seen in P. vivax and P. ovale infections. However, relapse-like P. vivax recurrences are probably being over-attributed to hypnozoite activation. Some of them might have an extra-vascular merozoite origin, making these recurrences recrudescences, not relapses. One newly recognised, non-hypnozoite, possible contributing source to recurrent peripheral P. vivax parasitemia is erythrocytic forms in bone marrow. P. vivax malaria cases in temperate areas often involve overwintering by hypnozoites, with relapses beginning the year after the mosquito bite. Reinfection means the parasite that caused the past infection was eliminated from the body but a new parasite was introduced. Reinfection cannot readily be distinguished from recrudescence, although recurrence of infection within two weeks of treatment for the initial infection is typically attributed to treatment failure. People may develop some immunity when exposed to frequent infections.
Climate change
Global climate change is likely to affect malaria transmission, but the degree of effect and the areas affected is uncertain. Greater rainfall in certain areas of India, and following an El Niño event is associated with increased mosquito numbers.
Since 1900 there has been substantial change in temperature and rainfall over Africa. However, factors that contribute to how rainfall results in water for mosquito breeding are complex, incorporating the extent to which it is absorbed into soil and vegetation for example, or rates of runoff and evaporation. Recent research has provided a more in-depth picture of conditions across Africa, combining a malaria climatic suitability model with a continental-scale model representing real-world hydrological processes.
Pathophysiology


Malaria infection develops via two phases: one that involves the liver (exoerythrocytic phase), and one that involves red blood cells, or erythrocytes (erythrocytic phase). When an infected mosquito pierces a person's skin to take a blood meal, sporozoites in the mosquito's saliva enter the bloodstream and migrate to the liver where they infect hepatocytes, multiplying asexually and asymptomatically for a period of 8–30 days.
After a potential dormant period in the liver, these organisms differentiate to yield thousands of merozoites, which, following rupture of their host cells, escape into the blood and infect red blood cells to begin the erythrocytic stage of the life cycle. The parasite escapes from the liver undetected by wrapping itself in the cell membrane of the infected host liver cell.
Within the red blood cells, the parasites multiply further, again asexually, periodically breaking out of their host cells to invade fresh red blood cells. Several such amplification cycles occur. Thus, classical descriptions of waves of fever arise from simultaneous waves of merozoites escaping and infecting red blood cells.
Some P. vivax sporozoites do not immediately develop into exoerythrocytic-phase merozoites, but instead, produce hypnozoites that remain dormant for periods ranging from several months (7–10 months is typical) to several years. After a period of dormancy, they reactivate and produce merozoites. Hypnozoites are responsible for long incubation and late relapses in P. vivax infections, although their existence in P. ovale is uncertain.
The parasite is relatively protected from attack by the body's immune system because for most of its human life cycle it resides within the liver and blood cells and is relatively invisible to immune surveillance. However, circulating infected blood cells are destroyed in the spleen. To avoid this fate, the P. falciparum parasite displays adhesive proteins on the surface of the infected blood cells, causing the blood cells to stick to the walls of small blood vessels, thereby sequestering the parasite from passage through the general circulation and the spleen. The blockage of the microvasculature causes symptoms such as those in placental malaria. Sequestered red blood cells can breach the blood–brain barrier and cause cerebral malaria.
Genetic resistance
According to a 2005 review, due to the high levels of mortality and morbidity caused by malaria—especially the P. falciparum species—it has placed the greatest selective pressure on the human genome in recent history. Several genetic factors provide some resistance to it including sickle cell trait, thalassaemia traits, glucose-6-phosphate dehydrogenase deficiency, and the absence of Duffy antigens on red blood cells.
The impact of sickle cell trait on malaria immunity illustrates some evolutionary trade-offs that have occurred because of endemic malaria. Sickle cell trait causes a change in the haemoglobin molecule in the blood. Normally, red blood cells have a very flexible, biconcave shape that allows them to move through narrow capillaries; however, when the modified haemoglobin S molecules are exposed to low amounts of oxygen, or crowd together due to dehydration, they can stick together forming strands that cause the cell to distort into a curved sickle shape. In these strands, the molecule is not as effective in taking or releasing oxygen, and the cell is not flexible enough to circulate freely. In the early stages of malaria, the parasite can cause infected red cells to sickle, and so they are removed from circulation sooner. This reduces the frequency with which malaria parasites complete their life cycle in the cell. Individuals who are homozygous (with two copies of the abnormal haemoglobin beta allele) have sickle-cell anaemia, while those who are heterozygous (with one abnormal allele and one normal allele) experience resistance to malaria without severe anaemia. Although the shorter life expectancy for those with the homozygous condition would tend to disfavour the trait's survival, the trait is preserved in malaria-prone regions because of the benefits provided by the heterozygous form.
Liver dysfunction
Liver dysfunction as a result of malaria is uncommon and usually only occurs in those with another liver condition such as viral hepatitis or chronic liver disease. The syndrome is sometimes called malarial hepatitis. While it has been considered a rare occurrence, malarial hepatopathy has seen an increase, particularly in Southeast Asia and India. Liver compromise in people with malaria correlates with a greater likelihood of complications and death.
Diagnosis


Owing to the non-specific nature of the presentation of symptoms, diagnosis of malaria in non-endemic areas requires a high degree of suspicion, which might be elicited by any of the following: recent travel history, enlarged spleen, fever, low number of platelets in the blood, and higher-than-normal levels of bilirubin in the blood combined with a normal level of white blood cells. Reports in 2016 and 2017 from countries where malaria is common suggest high levels of over diagnosis due to insufficient or inaccurate laboratory testing.
Malaria is usually confirmed by the microscopic examination of blood films or by antigen-based rapid diagnostic tests (RDT). In some areas, RDTs must be able to distinguish whether the malaria symptoms are caused by Plasmodium falciparum or by other species of parasites since treatment strategies could differ for non-P. falciparum infections. Microscopy is the most commonly used method to detect the malarial parasite—about 165 million blood films were examined for malaria in 2010. Despite its widespread usage, diagnosis by microscopy suffers from two main drawbacks: many settings (especially rural) are not equipped to perform the test, and the accuracy of the results depends on both the skill of the person examining the blood film and the levels of the parasite in the blood. The sensitivity of blood films ranges from 75 to 90% in optimum conditions, to as low as 50%. Commercially available RDTs are often more accurate than blood films at predicting the presence of malaria parasites, but they are widely variable in diagnostic sensitivity and specificity depending on manufacturer, and are unable to tell how many parasites are present. However, incorporating RDTs into the diagnosis of malaria can reduce antimalarial prescription. Although RDT does not improve the health outcomes of those infected with malaria, it also does not lead to worse outcomes when compared to presumptive antimalarial treatment.
In regions where laboratory tests are readily available, malaria should be suspected, and tested for, in any unwell person who has been in an area where malaria is endemic. In areas that cannot afford laboratory diagnostic tests, it has become common to use only a history of fever as the indication to treat for malaria—thus the common teaching "fever equals malaria unless proven otherwise". A drawback of this practice is overdiagnosis of malaria and mismanagement of non-malarial fever, which wastes limited resources, erodes confidence in the health care system, and contributes to drug resistance. Although polymerase chain reaction-based tests have been developed, they are not widely used in areas where malaria is common as of 2012, due to their complexity.
Classification
Malaria is classified into either "severe" or "uncomplicated" by the World Health Organization (WHO). It is deemed severe when any of the following criteria are present, otherwise it is considered uncomplicated.
- Decreased consciousness
- Significant weakness such that the person is unable to walk
- Inability to feed
- Two or more convulsions
- Low blood pressure (less than 70 mmHg in adults and 50 mmHg in children)
- Breathing problems
- Circulatory shock
- Kidney failure or haemoglobin in the urine
- Bleeding problems, or hemoglobin less than 50 g/L (5 g/dL)
- Pulmonary oedema
- Blood glucose less than 2.2 mmol/L (40 mg/dL)
- Acidosis or lactate levels of greater than 5 mmol/L
- A parasite level in the blood of greater than 100,000 per microlitre (µL) in low-intensity transmission areas, or 250,000 per µL in high-intensity transmission areas
Cerebral malaria is defined as a severe P. falciparum-malaria presenting with neurological symptoms, including coma (with a Glasgow coma scale less than 11, or a Blantyre coma scale less than 3), or with a coma that lasts longer than 30 minutes after a seizure.
Various types of malaria have been called by the names below:
Name | Pathogen | Notes |
---|---|---|
algid malaria | Plasmodium falciparum | severe malaria affecting the cardiovascular system and causing chills and circulatory shock |
bilious malaria | Plasmodium falciparum | severe malaria affecting the liver and causing vomiting and jaundice |
cerebral malaria | Plasmodium falciparum | severe malaria affecting the cerebrum |
congenital malaria | various plasmodia | plasmodium introduced from the mother via the fetal circulation |
falciparum malaria, Plasmodium falciparum malaria, pernicious malaria | Plasmodium falciparum | |
ovale malaria, Plasmodium ovale malaria | Plasmodium ovale | |
quartan malaria, malariae malaria, Plasmodium malariae malaria | Plasmodium malariae | paroxysms every fourth day (quartan), counting the day of occurrence as the first day |
quotidian malaria | Plasmodium falciparum, Plasmodium vivax, Plasmodium knowlesi | paroxysms daily (quotidian) |
tertian malaria | Plasmodium falciparum, Plasmodium ovale, Plasmodium vivax | paroxysms every third day (tertian), counting the day of occurrence as the first |
transfusion malaria | various plasmodia | plasmodium introduced by blood transfusion, needle sharing, or needlestick injury |
vivax malaria, Plasmodium vivax malaria | Plasmodium vivax |
Prevention

Methods used to prevent malaria include medications, mosquito elimination and the prevention of bites. As of 2020, there is one vaccine for malaria (known as RTS,S) which is licensed for use. The presence of malaria in an area requires a combination of high human population density, high anopheles mosquito population density and high rates of transmission from humans to mosquitoes and from mosquitoes to humans. If any of these is lowered sufficiently, the parasite eventually disappears from that area, as happened in North America, Europe, and parts of the Middle East. However, unless the parasite is eliminated from the whole world, it could re-establish if conditions revert to a combination that favors the parasite's reproduction. Furthermore, the cost per person of eliminating anopheles mosquitoes rises with decreasing population density, making it economically unfeasible in some areas.
Prevention of malaria may be more cost-effective than treatment of the disease in the long run, but the initial costs required are out of reach of many of the world's poorest people. There is a wide difference in the costs of control (i.e. maintenance of low endemicity) and elimination programs between countries. For example, in China—whose government in 2010 announced a strategy to pursue malaria elimination in the Chinese provinces—the required investment is a small proportion of public expenditure on health. In contrast, a similar programme in Tanzania would cost an estimated one-fifth of the public health budget.
In areas where malaria is common, children under five years old often have anaemia, which is sometimes due to malaria. Giving children with anaemia in these areas preventive antimalarial medication improves red blood cell levels slightly but does not affect the risk of death or need for hospitalisation.
Mosquito control

Vector control refers to methods used to decrease malaria by reducing the levels of transmission by mosquitoes. For individual protection, the most effective insect repellents are based on DEET or picaridin. However, there is insufficient evidence that mosquito repellents can prevent malaria infection. Insecticide-treated mosquito nets (ITNs) and indoor residual spraying (IRS) are effective, have been commonly used to prevent malaria, and their use has contributed significantly to the decrease in malaria in the 21st century. ITNs and IRS may not be sufficient to completely eliminate the disease as these interventions depend on how many people use nets, how many gaps in insecticide there are (low coverage areas), if people are not protected when outside of the home, and an increase in mosquitoes that are resistant to insecticides. Modifications to people's houses to prevent mosquito exposure may be an important long term prevention measure.
Insecticide treated nets
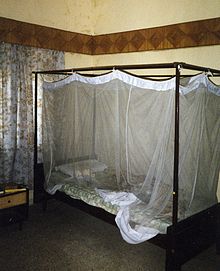
Mosquito nets help keep mosquitoes away from people and reduce infection rates and transmission of malaria. Nets are not a perfect barrier and are often treated with an insecticide designed to kill the mosquito before it has time to find a way past the net. Insecticide-treated nets are estimated to be twice as effective as untreated nets and offer greater than 70% protection compared with no net. Between 2000 and 2008, the use of ITNs saved the lives of an estimated 250,000 infants in Sub-Saharan Africa. About 13% of households in Sub-Saharan countries owned ITNs in 2007 and 31% of African households were estimated to own at least one ITN in 2008. In 2000, 1.7 million (1.8%) African children living in areas of the world where malaria is common were protected by an ITN. That number increased to 20.3 million (18.5%) African children using ITNs in 2007, leaving 89.6 million children unprotected and to 68% African children using mosquito nets in 2015. Most nets are impregnated with pyrethroids, a class of insecticides with low toxicity. They are most effective when used from dusk to dawn. It is recommended to hang a large "bed net" above the center of a bed and either tuck the edges under the mattress or make sure it is large enough such that it touches the ground. ITN is beneficial towards pregnancy outcomes in malaria-endemic regions in Africa but more data is needed in Asia and Latin America.
In areas of high malaria resistance, piperonyl butoxide combined with pyrethroids in ITN is effective in reducing malaria infection rates.
Indoor residual spraying
Indoor residual spraying is the spraying of insecticides on the walls inside a home. After feeding, many mosquitoes rest on a nearby surface while digesting the bloodmeal, so if the walls of houses have been coated with insecticides, the resting mosquitoes can be killed before they can bite another person and transfer the malaria parasite. As of 2006, the World Health Organization recommends 12 insecticides in IRS operations, including DDT and the pyrethroids cyfluthrin and deltamethrin. This public health use of small amounts of DDT is permitted under the Stockholm Convention, which prohibits its agricultural use. One problem with all forms of IRS is insecticide resistance. Mosquitoes affected by IRS tend to rest and live indoors, and due to the irritation caused by spraying, their descendants tend to rest and live outdoors, meaning that they are less affected by the IRS. It is uncertain whether the use of IRS together with ITN is effective in reducing malaria cases due to wide geographical variety of malaria distribution, malaria transmission, and insecticide resistance.
Housing modifications
Housing is a risk factor for malaria and modifying the house as a prevention measure may be a sustainable strategy that does not rely on the effectiveness of insecticides such as pyrethroids. The physical environment inside and outside the home that may improve the density of mosquitoes are considerations. Examples of potential modifications include how close the home is to mosquito breeding sites, drainage and water supply near the home, availability of mosquito resting sites (vegetation around the home), the proximity to live stock and domestic animals, and physical improvements or modifications to the design of the home to prevent mosquitoes from entering.
Other mosquito control methods
People have tried a number of other methods to reduce mosquito bites and slow the spread of malaria. Efforts to decrease mosquito larvae by decreasing the availability of open water where they develop, or by adding substances to decrease their development, are effective in some locations. Electronic mosquito repellent devices, which make very high-frequency sounds that are supposed to keep female mosquitoes away, have no supporting evidence of effectiveness. There is a low certainty evidence that fogging may have an effect on malaria transmission. Larviciding by hand delivery of chemical or microbial insecticides into water bodies containing low larval distribution may reduce malarial transmission. There is insufficient evidence to determine whether larvivorous fish can decrease mosquito density and transmission in the area.
Medications
There are a number of medications that can help prevent or interrupt malaria in travellers to places where infection is common. Many of these medications are also used in treatment. In places where Plasmodium is resistant to one or more medications, three medications—mefloquine, doxycycline, or the combination of atovaquone/proguanil (Malarone)—are frequently used for prevention. Doxycycline and the atovaquone/proguanil are better tolerated while mefloquine is taken once a week. Areas of the world with chloroquine-sensitive malaria are uncommon. Antimalarial mass drug administration to an entire population at the same time may reduce the risk of contracting malaria in the population.
The protective effect does not begin immediately, and people visiting areas where malaria exists usually start taking the drugs one to two weeks before they arrive, and continue taking them for four weeks after leaving (except for atovaquone/proguanil, which only needs to be started two days before and continued for seven days afterward). The use of preventive drugs is often not practical for those who live in areas where malaria exists, and their use is usually given only to pregnant women and short-term visitors. This is due to the cost of the drugs, side effects from long-term use, and the difficulty in obtaining antimalarial drugs outside of wealthy nations. During pregnancy, medication to prevent malaria has been found to improve the weight of the baby at birth and decrease the risk of anaemia in the mother. The use of preventive drugs where malaria-bearing mosquitoes are present may encourage the development of partial resistance.
Giving antimalarial drugs to infants through intermittent preventive therapy can reduce the risk of having malaria infection, hospital admission, and anaemia.
Mefloquine is more effective than sulfadoxine-pyrimethamine in preventing malaria for HIV-negative pregnant women. Cotrimoxazole is effective in preventing malaria infection and reduce the risk of getting anaemia in HIV-positive women. Giving sulfadoxine-pyrimethamine for three or more doses as intermittent preventive therapy is superior than two doses for HIV-positive women living in malaria-endemic areas.
Prompt treatment of confirmed cases with artemisinin-based combination therapies (ACTs) may also reduce transmission.
Others
Community participation and health education strategies promoting awareness of malaria and the importance of control measures have been successfully used to reduce the incidence of malaria in some areas of the developing world. Recognising the disease in the early stages can prevent it from becoming fatal. Education can also inform people to cover over areas of stagnant, still water, such as water tanks that are ideal breeding grounds for the parasite and mosquito, thus cutting down the risk of the transmission between people. This is generally used in urban areas where there are large centers of population in a confined space and transmission would be most likely in these areas. Intermittent preventive therapy is another intervention that has been used successfully to control malaria in pregnant women and infants, and in preschool children where transmission is seasonal.
Treatment

Malaria is treated with antimalarial medications; the ones used depends on the type and severity of the disease. While medications against fever are commonly used, their effects on outcomes are not clear. Providing free antimalarial drugs to households may reduce childhood deaths when used appropriately. Programmes which presumptively treat all causes of fever with antimalarial drugs may lead to overuse of antimalarials and undertreat other causes of fever. Nevertheless, the use of malaria rapid-diagnostic kits can help to reduce over-usage of antimalarials.
Uncomplicated malaria
Simple or uncomplicated malaria may be treated with oral medications. Artemisinin drugs are effective and safe in treating uncomplicated malaria. Artemisinin in combination with other antimalarials (known as artemisinin-combination therapy, or ACT) is about 90% effective when used to treat uncomplicated malaria. The most effective treatment for P. falciparum infection is the use of ACT, which decreases resistance to any single drug component. Artemether-lumefantrine (six-dose regimen) is more effective than the artemether-lumefantrine (four-dose regimen) or other regimens not containing artemisinin derivatives in treating falciparum malaria. Another recommended combination is dihydroartemisinin and piperaquine. Artemisinin-naphthoquine combination therapy showed promising results in treating falciparum malaria. However, more research need to establish its efficacy as a reliable treatment. Artesunate plus mefloquine performs better than mefloquine alone in treating uncomplicated falciparum malaria in low transmission settings. There is limited data to show atovaquone-proguanil is more effective than chloroquine, amodiaquine, and mefloquine in treating falciparum malaria. Azithromycin monotherapy or combination therapy has not shown effectiveness in treating plasmodium or vivax malaria. Amodiaquine plus sulfadoxine-pyrimethamine may achieve less treatment failures when compared to sulfadoxine-pyrimethamine alone in uncomplicated falciparum malaria. There is insufficient data on chlorproguanil-dapsone in treating uncomplicated falciparum malaria. The addition of primaquine with artemisinin-based combination therapy for falciparum malaria reduces its transmission at day 3-4 and day 8 of infection. Sulfadoxine-pyrimethamine plus artesunate is better than sulfadoxine-pyrimethamine plus amodiaquine in controlling treatment failure at day 28. However, the latter