Leukemia, Chronic Myeloid
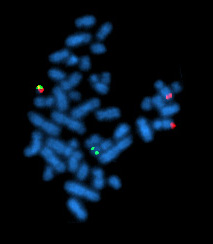
A number sign (#) is used with this entry because chronic myeloid leukemia is most frequently caused by a translocation between chromosomes 22 and 9, creating a BCR/ABL fusion gene encoding a tyrosine kinase (see 151410).
Clinical FeaturesChronic myeloid leukemia (CML) is a clonal myeloproliferative disorder of a pluripotent stem cell with a specific cytogenetic abnormality, the Philadelphia chromosome (Ph), involving myeloid, erythroid, megakaryocytic, B lymphoid, and sometimes T lymphoid cells, but not marrow fibroblasts. Silver (2003) reviewed the hematologic and clinical aspects of chronic myeloid leukemia. Geary (2000) presented a historical review of CML.
CML has a biphase or triphase clinical course (Medina et al., 2003). Approximately 90% of patients are diagnosed in the chronic phase, but the disease eventually evolves to a blastic phase unless successfully treated. Approximately two-thirds of patients manifest an accelerated phase. A distinct feature of disease progression is the appearance of additional cytogenetic abnormalities in the Ph-positive cells. This phenomenon, known as clonal evolution, frequently involves a second Ph, trisomy of chromosome 8, and isochromosome 17 and other abnormalities of chromosome 17 (Kantarjian et al., 1988), although other abnormalities have been described. Clonal evolution is considered a criterion of accelerated phase, although when it represents the only criterion of transformation, it is associated with a better prognosis than other criteria of accelerated phase (Cortes et al., 2003).
Sawyers (1999) reviewed the clinical aspects of chronic myeloid leukemia.
InheritanceLillicrap and Sterndale (1984) reported 3 cases of CML in 3 successive generations with a myeloproliferative disorder in a 4th member of the kindred.
CytogeneticsNowell and Hungerford (1960) identified the Philadelphia chromosome of chronic myeloid leukemia (a G group chromosome with part of its long arm missing). It was called the Philadelphia chromosome because it was thought to be useful to follow the practice of hemoglobinologists and name anomalous chromosomes after the city of discovery. It was presumed to be a deleted chromosome 21, the same chromosome as that which is trisomic in Down syndrome (190685). The elevated alkaline phosphatase activity in Down syndrome and depressed activity in CML was viewed as consistent with this interpretation. Using the improved definition provided by 'banding' methods, Rowley (1973) showed that in fact there is a translocation of the distal part of chromosome 22 (not 21) onto another chromosome, usually 9q.
De Klein et al. (1982) demonstrated that the Abelson oncogene (ABL; 189980) is translocated from chromosome 9 to chromosome 22 in the formation of the Philadelphia chromosome. This indicated that the translocation is reciprocal and suggested a role for the ABL gene in the generation of CML. Not only is the ABL oncogene translocated from 9q to 22 but the SIS oncogene (190040) is presumably translocated from 22 to 9 since it is situated distal to the breakpoint that creates the Philadelphia chromosome (Swan et al., 1982). What is involved in the variant 22q- Philadelphia chromosomes with translocations to other chromosomes? Some 18 different ones were found by Mittelman and Levan (1978). Do all these other chromosomes contribute oncogenes to the 22q- chromosome? Does the translocation of SIS to the recipient chromosome play some role in the usual CML and the variant forms? These were questions raised by Klein (1983), who also asked, Will the microevolutionary process that leads tumor cells towards increased independence from host control, usually referred to as tumor progression, turn out to depend on the sequential activation of multiple oncogenes by genetic rearrangement?
Prakash and Yunis (1984) located the breakpoints in CML to subbands 22q11.21 and 9q34.1. Although the position of the breakpoint in chromosome 9 is quite variable, the breakpoint in chromosome 22 is clustered in an area called BCR for 'breakpoint cluster region.' Shtivelman et al. (1985) referred to BCR as a gene and stated that the ABL oncogene is transferred 'into the BCR gene of chromosome 22.' They found that an 8-kb RNA specific to CML is a fused transcript of the 2 genes. The fused protein is presumably involved in the malignant process. The protein has BCR information at its amino terminus and retains most but not all of the normal ABL protein sequences. Since the breakpoint in 9 may be as much as 30 or 40 kb 5-prime to ABL, a large amount must be 'looped out' in the fusion process. The fusion protein has tyrosine kinase activity.
About 10% of patients with acute lymphocytic leukemia (ALL) have the translocation t(9;22)(q34;q11) indistinguishable from that of CML. Erikson et al. (1986), however, found in 3 of 5 such cases of ALL that the BCR region was not involved and that the 22q11 chromosome breakpoint was proximal (5-prime) to the BCR region. Furthermore, the BCR and ABL transcripts were of normal size in an ALL line carrying the t(9;22) translocation. The breakpoints of the t(9;22) CML, the t(9;22) of ALL, and the t(8;22) of Burkitt lymphoma fall into 22q11 and are cytologically indistinguishable. By chromosomal in situ hybridization, however, they can be distinguished (Emanuel et al., 1984; Erikson et al., 1986). To this experience, Griffin et al. (1986) added observations on t(11;22), both constitutional and tumor-related (see 133450). In the hybrid gene of CML, the BCR contribution is 5-prime to the ABL contribution. In the creation of the gene, splicing can occur across an interval as great as 100 kb. The product of the BCR/ABL hybrid gene is a 210-kD protein. It was suggested that the hybrid gene and its product protein be designated PHL.
Ganesan et al. (1986) found that the BCR gene was rearranged in 7 cases of Philadelphia chromosome-negative CML. In 5 cases hematologic findings were indistinguishable from those of patients with the Philadelphia chromosome.
Mes-Masson et al. (1986) isolated overlapping cDNA clones defining the complete coding region for the hybrid protein generated from the ABL and BCR genes.
Schaefer-Rego et al. (1987) found that the breakpoints in 8 of 9 patients in blast crisis were in the 3-prime portion of BCR, whereas the breakpoints in 17 patients in the chronic phase were clustered in the 5-prime portion.
Mills et al. (1988) found a striking correlation between the site of the breakpoint within BCR and the length of time between presentation and onset of acute phase in CML patients: on average, patients with a 5-prime breakpoint had a 4-fold longer chronic phase than those with a 3-prime breakpoint. The median times were 203 weeks versus 52 weeks. Grossman et al. (1989) also presented evidence consistent with but not proving a relationship between the site of the breakpoint in BCR and the length of the clinical course before onset of blast crisis. Patients with 3-prime breakpoints progressed to acute disease after a shorter period (average 36.6 months) than did patients with 5-prime breakpoints (average 56.1 months), although the difference was not statistically significant.
Demiroglu et al. (2001) described 2 patients with a clinical and hematologic diagnosis of CML in chronic phase who had an acquired t(8;22)(p11;q11). They confirmed that both patients were negative for a BCR-ABL fusion gene and that both had an in-frame mRNA fusion between BCR exon 4 and FGFR1 (136350) exon 9. Thus, a BCR-FGFR1 fusion may occur in patients with apparently typical CML. The possibility of successful treatment with specific FGFR1 inhibitors was suggested.
Saglio et al. (2002) found that a patient with a typical form of chronic myeloid leukemia carried a large deletion on the derivative chromosome 9q+ and an unusual BCR-BCL transcript characterized by the insertion, between BCR exon 14 and ABL exon 2, of 126 bp derived from a region located on chromosome 9, 1.4 Mb 5-prime to ABL. This sequence was contained in a bacterial artificial chromosome (BAC), which in FISH experiments on normal metaphases was found to detect, in addition to the predicted clear signal at 9q34, a faint but distinct signal at 22q11.2, where the BCR gene is located, suggesting the presence of a large region of homology between the 2 chromosome regions. A BLAST analysis of the particular BAC sequence against the entire human genome revealed the presence of a stretch of homology, about 76 kb long, located approximately 150 kb 3-prime to the BCR gene, and containing the 126-bp insertion sequence. Evolutionary studies using FISH identified the region as a duplicon, which transposed from the region orthologous to human 9q34 to chromosome 22 after the divergence of orangutan from the human-chimpanzee-gorilla common ancestor about 14 million years ago. Saglio et al. (2002) noted that sequence analyses reported as part of the Human Genome Project had disclosed an unpredicted extensive segmental duplication in the human genome, and the impact of duplicons in triggering genomic disorders is becoming more and more apparent. The discovery of a large duplicon relatively close to the ABL and BCR genes and the finding that the 126-bp insertion is very close to the duplicon at 9q34 open the question of the possible involvement of the duplicon in the formation of the Philadelphia chromosome translocation.
Goldman and Melo (2003) tabulated 15 cytogenetic abnormalities leading to the expression of deregulated tyrosine kinases in chronic myeloproliferative disorders, beginning with BCR-ABL, which can cause either CML or ALL.
PathogenesisAlong with clinical aspects of chronic myeloid leukemia, Sawyers (1999) reviewed the molecular aspects of its pathogenesis.
Goldman and Melo (2003) summarized advances in knowledge related to the pathogenesis of CML.
Ito et al. (2010) used mouse models of CML to show that disease progression is regulated by the Musashi-Numb signaling axis. Specifically, Ito et al. (2010) found that the chronic phase of CML is marked by high levels of Numb (603728) expression whereas the blast crisis phase has low levels of Numb expression, and that ectopic expression of Numb promotes differentiation and impairs advanced-phase disease in vivo. As a possible explanation for the decreased levels of Numb in the blast crisis phase, Ito et al. (2010) showed that Nup98-Hoxa9 (see 601021), an oncogene associated with blast crisis CML, can trigger expression of the RNA-binding protein Musashi-2 (MSI2; 607897), which in turn represses Numb. Notably, loss of Msi2 restores Numb expression and significantly impairs the development and propagation of blast crisis CML in vitro and in vivo. Finally, Ito et al. (2010) found that Msi2 expression is not only highly upregulated during human CML progression but is also an early indicator of poor prognosis.
Abraham et al. (2016) used proteomics, transcriptomics, and network analyses to show that in human leukemic stem cells (LScs), aberrantly expressed proteins in both imatinib-responder and -nonresponder patients are modulated in concert with p53 (191170) and c-MYC (190080) regulation. Perturbation of both p53 and c-MYC, and not BCR-ABL itself, leads to synergistic cell kill, differentiation, and near elimination of transplantable human LSCs in mice, while sparing normal hematopoietic stem cells. Abraham et al. (2016) concluded that this unbiased systems approach targeting connected nodes exemplified a novel precision medicine strategy providing evidence that LSCs can be eradicated.
Clinical ManagementSawyers (1999) reviewed therapeutic choices available in chronic myeloid leukemia based on new knowledge of molecular aspects of its pathogenesis.
Because tyrosine kinase activity is essential to the transforming function of BCR-ABL, Druker et al. (2001) reasoned that an inhibitor of the kinase may be an effective treatment for CML. They found that indeed a tyrosine kinase inhibitor (STI571) was well tolerated and had significant antileukemic activity in patients with CML in whom treatment with standard chemotherapy had failed. This experience demonstrated the potential for the development of anticancer drugs based on the specific molecular abnormality present in a human cancer.
Druker et al. (2001) found that the same BCR-ABL tyrosine kinase inhibitor was well tolerated and had substantial activity in the blast crises of CML and in Philadelphia-chromosome-positive ALL. The response was less satisfactory in the ALL group. Goldman and Melo (2001) discussed the likely role of the kinase inhibitor in relation to other forms of therapy for CML. Goldman and Melo (2001) also illustrated the likely mode of action of STI571.
Imatinib (Gleevec, Novartis, Basel, Switzerland), formerly referred to as STI571, was approved by the Food and Drug Administration in May 2001 for the treatment of CML that is refractory to interferon therapy and in February 2002 for the treatment of gastrointestinal stromal tumors (606764), which can be caused by mutations in the KIT gene (164920) (Savage and Antman, 2002). In both cases the agent works as an inhibitor of specific protein tyrosine kinases.
Barbany et al. (2002) described a patient in whom complete molecular response was achieved with no evidence of BCR-ABL mRNA 6 months after treatment with imatinib was begun. The patient was a 58-year-old man with Philadelphia chromosome-positive CML in chronic phase. He was initially treated with interferon alfa followed by intensive chemotherapy plus granulocyte colony-stimulating factor, which allowed the successful collection of Ph-negative blood stem cells. Subsequently, he underwent autologous blood stem cell transplantation. Cytogenetic relapse was detected 34 months after transplantation in a routine blood marrow examination. Treatment with interferon alfa was reinstated but had to be discontinued because of side effects (depression) with no signs of cytogenetic response. Treatment with imatinib was then initiated.
Olavarria et al. (2002) described a male patient who had a relapse to chronic phase after stem cell transplantation for CML and did not benefit from treatment with donor lymphocyte infusion. After 6 months of therapy with STI571, the patient had a rapid, complete hematologic response, and complete restoration of donor-type hematopoiesis, with 100% female marrow metaphases, although RT-PCR still detected BCR-ABL transcripts in the blood at low level.
Goldman and Melo (2003) discussed the therapeutic implications of advances in knowledge of the biology of CML.
Zhao et al. (2009) demonstrated that the loss of Smoothened (Smo; 601500), an essential component of the hedgehog pathway (see 600725), impairs hematopoietic stem cell renewal and decreases induction of CML by the BCR-ABL1 oncoprotein (see 151410). Loss of Smo causes depletion of CML stem cells, which propagate the leukemia, whereas constitutively active Smo augments CML stem cell number and accelerates disease. As a possible mechanism for Smo action, Zhao et al. (2009) showed that the cell fate determinant Numb (603728), which depletes CML stem cells, is increased in the absence of Smo activity. Furthermore, pharmacologic inhibition of hedgehog signaling impairs not only the propagation of CML driven by wildtype BCR-ABL1, but also the growth of imatinib-resistant mouse and human CML. Zhao et al. (2009) concluded that hedgehog pathway activity is required for maintenance of normal and neoplastic stem cells of the hematopoietic system and raised the possibility that the drug resistance and disease recurrence associated with imatinib treatment of CML might be avoided by targeting this essential stem cell maintenance pathway.
Resistance of Bcr-Abl-positive leukemic stem cells (LSCs) to imatinib treatment in patients with CML can cause relapse of disease and might be the origin for emerging drug-resistant clones. Dierks et al. (2008) identified Smo as a drug target in Bcr-Abl-positive LSCs. They showed that Hedgehog signaling is activated in LSCs through upregulation of Smo. While nullity for Smo does not affect long-term reconstitution of regular hematopoiesis, the development of retransplantable Bcr-Abl-positive leukemias was abolished in the absence of Smo expression. Pharmacologic Smo inhibition reduced LSCs in vivo and enhanced time to relapse after end of treatment. Dierks et al. (2008) postulated that Smo inhibition might be an effective treatment strategy to reduce the LSC pool in CML.
Prost et al. (2015) showed that the residual CML leukemia stem cell (LSC) pool can be gradually purged by the glitazones, antidiabetic drugs that are agonists of peroxisome proliferator-activated receptor-gamma (PPAR-gamma; 601487). The authors found that activation of PPAR-gamma by the glitazones decreases expression of STAT5 (see 601511) and its downstream targets HIF2-alpha (603349) and CITED2 (602937), which are key guardians of the quiescence and stemness of CML LSCs. When pioglitazone was given temporarily to 3 CML patients in chronic residual disease in spite of continuous treatment with imatinib, all of them achieved sustained complete molecular response, up to 4.7 years after withdrawal of pioglitazone. Prost et al. (2015) concluded that this suggested that clinically relevant cancer eradication may become a generally attainable goal by combination therapy that erodes the cancer stem cell pool.
Molecular GeneticsAssociations with Drug Resistance
Clinical studies with the Abl tyrosine kinase inhibitor STI571 in CML demonstrated that many patients with advanced-stage disease respond initially but then relapse. Through biochemical and molecular analysis of clinical material, Gorre et al. (2001) found that the drug resistance was associated with a reactivation of BCR-ABL signal transduction in all cases examined. In 6 of 9 patients, resistance was associated with a single amino acid substitution in a threonine residue of the Abl kinase domain known to form a critical hydrogen bond with the drug. This T351I substitution (189980.0001) was sufficient to confer STI571 resistance in a reconstitution experiment. In 3 patients, resistance was associated with progressive BCR-ABL gene amplification. Gorre et al. (2001) concluded that their studies provided evidence that genetically complex cancers retain dependence on an initial oncogenic event and suggest a strategy for identifying inhibitors of STI571 resistance.
Azam et al. (2003) stated that sequencing of the BCR-ABL gene in patients who relapsed after STI571 chemotherapy revealed a limited set of kinase domain mutations that mediate drug resistance. To obtain a more comprehensive survey of the amino acid substitutions that confer STI571 resistance, they performed an in vitro screen of randomly mutagenized BCR-ABL and recovered all the major mutations previously identified in patients and numerous others that illuminated novel mechanisms of acquired drug resistance. Structural modeling implied that a novel class of variants acts allosterically to destabilize the autoinhibited conformation of the ABL kinase, to which STI571 preferentially binds. The authors concluded that this screening strategy is a paradigm applicable to a growing list of target-directed anticancer agents and provides a means of anticipating the drug-resistant amino acid substitutions that are likely to be clinically problematic.
Goldman and Melo (2003) tabulated 19 BCR-ABL point mutations associated with resistance to the therapeutic effects of the anti-tyrosine-kinase agent, imatinib.
Hantschel et al. (2007) identified the BTK tyrosine kinase (300300) and TEC kinase (600583) as major binders of the tyrosine kinase inhibitor dasatinib, which is used for treatment of BCR/ABL-positive CML. Dasatinib did not bind ITK (186973). Mutations of the gatekeeper residue in the BTK and TEC gene conferred resistance to dasatinib in cultured cells. Both BTK and TEC mutations were structurally homologous to the T315I mutation in the ABL gene that confers resistance to imatinib. Analysis of mast cells derived from Btk-deficient mice suggested that inhibition of Btk by dasatinib may be responsible for the observed reduction in histamine release upon dasatinib treatment. Dasatinib inhibited histamine release in primary human basophils and secretion of proinflammatory cytokines in immune cells. The findings suggested that dasatinib may have immunosuppressive side effects.
Genetic Modifiers
Peeters et al. (1997) identified a t(9;12)(p24;p13) translocation in a patient with early pre-B acute lymphoid leukemia and a t(9;15;12)(p24;q15;p13) translocation in a patient with atypical chronic myelogenous leukemia in transformation. Both changes involved the ETV6 gene (600618) at chromosome 12p13 and the JAK2 gene (147796) at chromosome 9p24. In each case different fusion mRNAs were found, with only 1 resulting in a chimeric protein consisting of the oligomerization domain of ETV6 and the protein tyrosine kinase domain of JAK2.
In bone marrow cells derived from 8 (9.41%) of 85 unrelated patients with CML during blast crisis transformation, Zhang et al. (2008) identified heterozygosity for a somatic leu359-to-val (L359V) mutation in the GATA2 gene (137295). This mutation was not present during the chronic phase of the disease. One additional patient with blast crisis and eosinophilia had an in-frame 18-bp deletion in the GATA2 gene. Both mutations were located within or close to the N terminus of the ZF2 domain, which is responsible for DNA binding, self-association, and heterodimerization. Neither mutation was detected in 200 healthy controls or in 233 patients with other forms of blood cancer. The patients with GATA2 mutations showed a poorer prognosis compared to other CML patients in blast crisis. Functional expression studies showed that the L359V mutation resulted in a gain-of-function effect with increased activity, whereas the deletion resulted in decreased activity. The L359V mutation also enhanced the inhibitory effects on the activity of PU.1 (SPI1; 165170), a major regulator of myelopoiesis. Transduction of the GATA2 L359V mutant into HL-60 cells or BCR/ABL-harboring murine cells disturbed myelomonocytic differentiation/proliferation in vitro and in vivo, respectively. These data strongly suggest that GATA2 mutations play a role in acute myeloid transformation in a subset of CML patients.
Atypical Chronic Myeloid Leukemia
Atypical CML (aCML) shares clinical and laboratory features with CML but lacks the pathognomonic Philadelphia chromosome and the resulting BCR-ABL1 fusion, pointing to a different pathogenetic process. Using exome sequencing, Piazza et al. (2013) identified heterozygous somatic alterations of SETBP1 (611060) encoding a gly870 to ser (G870S) alteration in 2 of 8 aCMLs. Targeted resequencing detected SETBP1 mutations in 17 of 70 aCMLs (24.3%; 95% confidence interval = 16-35%); 16 of these mutations occurred between residues 858 and 871, within the SKI homologous region. Clinical information was available for 38 aCML cases, including 14 with SETBP1 mutations. SETBP1-mutated cases showed worse prognosis (median survival 22 vs 77 months) and presented with higher white blood cell counts at diagnosis (median of 81.0 vs 38.5 x 10(9) cells/l) compared to cases with wildtype SETBP1. No significant differences were observed in the number of peripheral blood blasts, age, hemoglobin concentration, platelet counts, or sex distribution. Piazza et al. (2013) concluded that SETBP1 mutations are present in approximately one quarter of aCML cases.
Other FeaturesAlthough CML is fundamentally a genetic disorder, it may also have environmental causes such as irradiation and chemical (e.g., benzene) exposure (Jacobs, 1989). Court Brown and Doll (1965) followed up more than 14,000 patients irradiated in the treatment of ankylosing spondylitis in British clinics between 1935 and 1954. A high frequency of Philadelphia-chromosome-positive CML was found. This is an example of a chromosomal change (specific translocation) being the common oncogenetic mechanism for various 'causes' which may include viral infection and chemicals in addition to ionizing radiation.
Animal ModelWhen the Philadelphia-chromosome-positive chronic myeloid leukemia-blast crisis cell line BV173 is injected into SCID mice, a disease process closely resembling that seen in leukemia patients results (Kamel-Reid et al., 1989). For example, BCR-ABL transcripts are detectable in bone marrow, spleen, peripheral blood, liver, and lungs. Skorski et al. (1994) found that systemic treatment of the leukemic mice with a 26-mer BCR-ABL antisense oligodeoxynucleotide induced disappearance of leukemic cells and a marked decrease in BCR-ABL mRNA in mouse tissues. Untreated mice or mice treated with a BCR-ABL sense oligodeoxynucleotide or a 6-base-mismatched antisense oligodeoxynucleotide were dead 8 to 13 weeks after leukemia cell injection; in marked contrast, mice treated with BCR-ABL antisense oligodeoxynucleotide died of leukemia 18 to 23 weeks after injection of leukemic cells. Findings were interpreted as indicating the in vivo effectiveness of an anticancer therapy based on antisense oligodeoxynucleotides targeting a tumor-specific gene.
To determine whether the P210(bcr/abl) hybrid protein can induce leukemia, Daley et al. (1990) infected murine bone marrow with a retrovirus encoding this protein and transplanted the bone marrow into irradiated syngeneic recipients. Transplant recipients developed several hematologic malignancies, prominent among which was a myeloproliferative syndrome closely resembling the chronic phase of human chronic myelogenous leukemia. Tumor tissue from diseased mice harbored the provirus encoding P210(bcr/abl).
Cancer is thought to arise from multiple genetic events that establish irreversible malignancy. A different mechanism might be present in certain leukemias initiated by a chromosomal translocation. Huettner et al. (2000) adopted a new approach to determine if ablation of the genetic abnormality is sufficient for reversion. They generated a conditional transgenic model of BCR-ABL-induced leukemia. The most common form of the product of the fusion gene, p210 BCR-ABL1, is found in more than 90% of patients with chronic myelogenous leukemia and in up to 15% of adult patients with de novo ALL. Efforts to establish a useful transgenic model had been hampered by embryonic lethality when the oncogene is expressed during embryogenesis, by reduced penetrance, or by extremely long latency. Huettner et al. (2000) used the 'knock-in' approach to induce leukemia by p190 BCR-ABL1 (Castellanos et al., 1997). Lethal leukemia developed within an acceptable time frame in all animals, and complete remission was achieved by suppression of BCR-ABL1 expression, even after multiple rounds of induction and reversion. The results demonstrated that BCR-ABL1 is required for both induction and maintenance of leukemia. The findings suggested that complete and lasting remissions can be achieved if the genetic abnormality is abolished or silenced before secondary mutations are acquired. The results have implications for therapies that directly target leukemia oncogenes, with a relevant example being the use of BCR-ABL1-specific tyrosine kinase inhibitors.
Wong et al. (2003) showed that p210 Bcr-Abl-expressing mouse bone marrow cells deficient in either the Il3 receptor-beta common chain (see 138981) or both the common chain and the beta chain were able to generate myeloproliferative disease, indicating that IL3 (147740) signal transduction is not essential for BCR-ABL-induced myeloproliferative disease.
Krause et al. (2006) found that mice lacking Cd44 (107269) were as susceptible as wildtype mice to murine CML after challenge with BCR-ABL virus. However, bone marrow cells from Cd44 -/- mice transduced with the virus showed defective homing to recipient bone marrow, resulting in decreased engraftment and reduced CML-like disease. In contrast, Cd44 was dispensable for induction of B-lymphoblastic leukemia-like disease. Krause et al. (2006) concluded that CD44 is required for leukemic stem cells that initiate CML.
Chen et al. (2009) found that Alox5 (152390)-null mice were resistant to the development of BCR/ABL-induced CML. Initial cellular studies showed that leukemia stem cells (LSCs) that had been transduced with and expressed BCR/ABL showed upregulation of Alox5, compared to cells that did not express BCR/ABL. Alox5 deficiency caused impairment of the function of LSCs by affecting differentiation, cell division, and survival of long-term LSCs. This resulted in a depletion of LSCs and a failure of CML development. In contrast, Alox5 deficiency did not impair the function of normal hematopoietic stem cells. Further studies indicated that an intact Alox5 pathway was essential for the induction of CML by BCR/ABL. Treatment of CML mice with a 5-LO inhibitor also impaired the function of LSCs by affecting the long-term LSCs, and prolonged survival. These results demonstrated that a specific target gene can be found in cancer stem cells and its inhibition can completely inhibit the function of these stem cells.